Beyond Mice Models: Exploring Human Aging Genetics with Dr. Joris Deelen on The VitaDAO Aging Science Podcast

Limitations of mice as model organism have been a recurring theme of the last few episodes. Nothing highlights this better than the human genetic data, since it often seems to disagree with the mouse data. In this podcast I discuss with Dr. Joris Deelen (@Joris_Deelen) discuss whether this is really true and what we can learn about aging from human genetic studies.
We both agree that the state of the art in his field "is quite depressing, [yet also] also quite interesting".
Joris Deelen – short bio
Joris Deelen obtained his PhD at the Leiden University Medical Center in The Netherlands in 2014 in the group of Prof. Dr. Eline Slagboom. In 2016, he joined the Max Planck Institute for Biology of Ageing as a postdoctoral researcher in the group of Prof. Dr. Linda Partridge, where he was promoted to independent Research Group Leader in 2020. The work in his group is mainly focused on the identification and functional characterisation of genetic variants linked to human longevity. To this end, they make use of the CRISPR/Cas9 system to generate transgenic cell lines and model organisms harbouring the identified variants and subsequently measure their functional effects in vitro and in vivo.
GWAS studies – our main tool to study the genetics of human longevity
GWAS stands for Genome-Wide Association Studies. These studies are a type of research method used in genetics, mainly for the identification of genetic variants associated with particular diseases. GWAS involves the examination of a genome-wide set of genetic variants in different individuals to see if any variant is associated with a trait or disease.
The process begins with the collection of DNA samples from many people who have a certain disease (cases) and from people who do not have the disease (controls). The DNA is then scanned for genetic markers, usually single-nucleotide polymorphisms (SNPs), small differences in the DNA sequence that occur between individuals. The frequency of these markers in the disease group is compared to the control group to find any significant associations.
Interestingly many times the variants found are not even in the protein-coding region of the gene, probably because such variants would be rare due to their high impact on protein function.
The major ways of running GWAS studies for longevity
There are different ways of analyzing the data that will yield slightly different interpretations. The major difference is in the outcome we study. Although we may use the umbrella term “longevity”, there is actually more than one longevity-related outcome of interest. We can study genes that predict survival to old age (as a binary variable), this is most similar to a classic case-control design. A different take on this method is using the whole range of lifespan values, which may increase power but will also include people who died of diseases not related to aging. Both these approaches suffer from one major limitation. We have to wait until a large portion of the cohort has died before we can apply them!
An alternative is to measure how genetic variants correlate with the lifespan of a participant’s parents. This will give a valid estimate for the longevity of each participant because longevity is heritable. Finally, as done by Joris and others, we can also look at healthspan as an outcome, aggregating data from multiple age-related diseases that participants might currently have – obviating the need for a full mortality analysis.
Missing heritability of aging
Genetic heritability estimates the proportion of variation, or differences, in a phenotypic trait in a population that is due to genetic variation between individuals within that population. While the heritability of lifespan is not very high, the heritability of extreme survivorship is considerably higher. As aging researchers we are more interested in extreme survival, e.g. people who become centenarians.
Regardless of which outcome you look at, it appears that GWAS studies fail to find all the variants that would account for the heritability of longevity predicted from twin studies. Joris and I discuss why this is the case in the podcast.
Similarly, the heritability of age-related diseases is much higher than the heritability of lifespan. Therefore, I believe, it might be possible to find novel variants associated with lifespan to close the heritability gap, at least somewhat.
Genetic variants: rare vs common
The term SNV stands for single nucleotide variants and SNP stands for single nucleotide polymorphisms. While there is a subtle difference between the two, for the purposes of this podcast we are dealing with small, single nucleotide changes at a specific position in the genome. These genetic variants, differences between people, can be then studied for their association with different traits like longevity.
Some variants are rarer than others. Common variants may be found in 1% of the population, while variants at below 1% are called rare. Ultra rare are found in fewer than 0.1% of the population and in some cases even unique variants may be of interest. Imagine you studied the genome of the oldest person in the world and found a unique variant in a “candidate” longevity gene like growth hormone. This would be obviously scientifically interesting.
The curious case of ApoE and how it compares with other variants
ApoE is a fascinating case study for the success and failure of GWAS studies. It is the best known and most robust genetic variant associated with longevity. It is by far the most robust one, as we both lament. Almost none of the other variants identified so far replicate in all human populations, except ApoE.
It has been shown that the ε2 (E2) allele is overrepresented in long-lived individuals compared to control groups, while the ε4 allele is underrepresented. The ε3 allele, being the most common, does not seem to have a strong effect either way. The E2 allele may be also linked to lower risk of Alzheimer’s disease and cardiovascular disease. Interestingly, ApoE genotype could be more important in people who eat a lot of cholesterol, i.e. cholesterol would be more risky if you have an E4 genotype.
In a way ApoE is also interesting because it breaks a common pattern seen in longevity GWAS. Usually variants are not protein altering, except for the ApoE alleles which do change the actual protein, giving rise to isoforms.
Finally, it is important to point out that ApoE by itself would not be enough to meaningfully extend human lifespan since we are looking at a small extension in absolute terms even in the base case. If I recall correctly Joris mentioned something on the order of 2 years. If we want to robustly extend human lifespan we need to discover gerotherapeutics that work in humans as well as does rapamycin in mice.
Alternative approaches and future directions for genetic studies
Using candidate gene approaches, where a candidate is defined based on animal data, e.g. “insulin signaling pathway”, rare or even unique variants enriched in long-lived populations are collected and then studied further and validated in animal models. The work Joris himself does or Vera Gorbunova did on SIRT6 falls into this category.
Most GWAS studies are performed in populations of European ancestry or in East Asian populations, whereas there is very little data from Africa, South America and many other regions. If we could diversify our datasets we might be able to identify novel variants specific to these populations and better confirm our so called “universal” variants.
Novel techniques including long read sequencing to study changes in structural variants and larger scale chromosomal changes as they related to aging. Even just a shift from array genotyping to standard whole genome sequencing could help to reveal novel longevity-related variants.
We also discssus collaborations with 23andme or other companies that sell commercial tests and how we could use this to increase the total sample size of available longevity GWAS studies. Here the limiting factor is that these companies may be unable to keep track of mortality statistics and/or did not inquire about parental longevity, the two key outcomes we would use in GWAS studies.
Another promising technique is mendelian randomization. We call it randomization because each person randomly inherits genetic variants from their parents. Using GWAS we then define a set of variants that are interesting (because they predict X) and then we ask if they are associated with longevity, which will also tell us whether X is causally associated with longevity.
One example would be asking whether genetically proxied risk for Alzheimer’s disease predicts lifespan, another would be to look at predicted LDL or iron levels. If a genetic variant is associated with higher levels of LDL cholesterol, and if the same variant is also associated with an increased risk of heart disease, one could infer that higher LDL cholesterol levels cause an increased risk of heart disease (this is indeed the case; Daghlas and Gill 2021). Below we discuss this approach for iron.
Iron and Aging – a case study for the usefulness of genetics
As a part-time iron researcher myself, I was pleased to discuss Joris’ work on iron, where he used mendelian randomization to test whether genetically predicted iron levels are related to measures of longevity. There is a plausible link between iron and aging. In fact, I have written an article on this topic not long ago, which while quite focused on supplementation, nutrition and blood testing, nevertheless provides a good review of the evidence on this topic: (https://novoslabs.com/iron-longevity-aging-cancer/)
“Iron is a mineral that’s essential to human health and life. Your body uses iron to generate the protein hemoglobin, which red blood cells use to carry oxygen from the lungs throughout the body, as well as the protein myoglobin, which provides oxygen to muscles. The body requires a careful balance of iron in just the right amounts: In studies, iron has presented as one of the few micronutrients that shows a pronounced U-shaped dose-benefit-response curve within a relatively narrow range of intakes or blood levels. This means that both too much and too little iron is harmful to your health.
Under normal circumstances, iron is so precious that nature did not come up with an active mechanism for the excretion of this metal. Bleeding and cell shedding are the major ways in which the body loses iron and modulates uptake. While iron is utilized by many enzymes, it is nonetheless dangerous to the body under certain circumstances. Unbound or free iron can catalyze oxidative stress and DNA damage through the so-called Fenton reaction.”
In this study (Timmers et al. 2020) Joris and colleagues first went back to the definition of longevity before even starting the analysis. Combining data from studies looking at parental lifespan, healthspan and long-lived participants they were able to validate and extend the list of significant genes found in GWAS studies. Having established that, they identified which so called quantitative trait loci (QTL) were colocalizing with the above GWAS variants. Since QTLs predict “quantitative” changes in some phenotype (e.g. height or cholesterol) they were able to put them together and test for enrichment of pathways that could affect these phenotypes. One pathway they found was “heme metabolism” and, as far as I understand, this then led them to perform full-scale mendelian randomization of iron traits, finding that lower iron was beneficial for longevity.
Although as a non-geneticist, I do not pretend to fully understand what they did, it is certainly good work based on plausible biology that has been solidly replicated (Daghlas and Gill 2021).
Studying accelerated aging (progerias)
We also talk about an often-controversial topic which is progerias. Some people study these syndromes since they appear to accelerate aging. During the podcast we speculate that there should be progeroid rare variants that produce less severe symptoms, which could teach us something about pro-longevity pathways (whose loss of function is harmful to lifespan).
However, there are many ways to shorten our lifespan genetically and fewer that will extend lifespan. Furthermore, the variants that shorten lifespan will do this in different ways, whereas there is only one way to live longer, which is by slowing aging or one of the major age-related diseases. You may call this the Anna Karenina principle applied to longevity if you will.
The host – brief bio
Kamil Pabis, MSc is an aging researcher and longevity advocate with several years of experience in the aging field that spans multiple countries. Among other projects, Kamil worked on long-lived dwarf mice in Austria, on mitochondrial disease and aging in the UK, and finally on the bioinformatics of aging in Germany and Singapore. Presently, he is involved in several projects related to science communication and translational aging research.

References and further reading
Daghlas, Iyas, and Dipender Gill. "Low‐density lipoprotein cholesterol and lifespan: A Mendelian randomization study." British Journal of Clinical Pharmacology 87.10 (2021): 3916-3924.
Timmers, Paul RHJ, et al. "Multivariate genomic scan implicates novel loci and haem metabolism in human ageing." Nature communications 11.1 (2020): 3570.
Daghlas, Iyas, and Dipender Gill. "Genetically predicted iron status and life expectancy." Clinical Nutrition 40.4 (2021): 2456-2459.
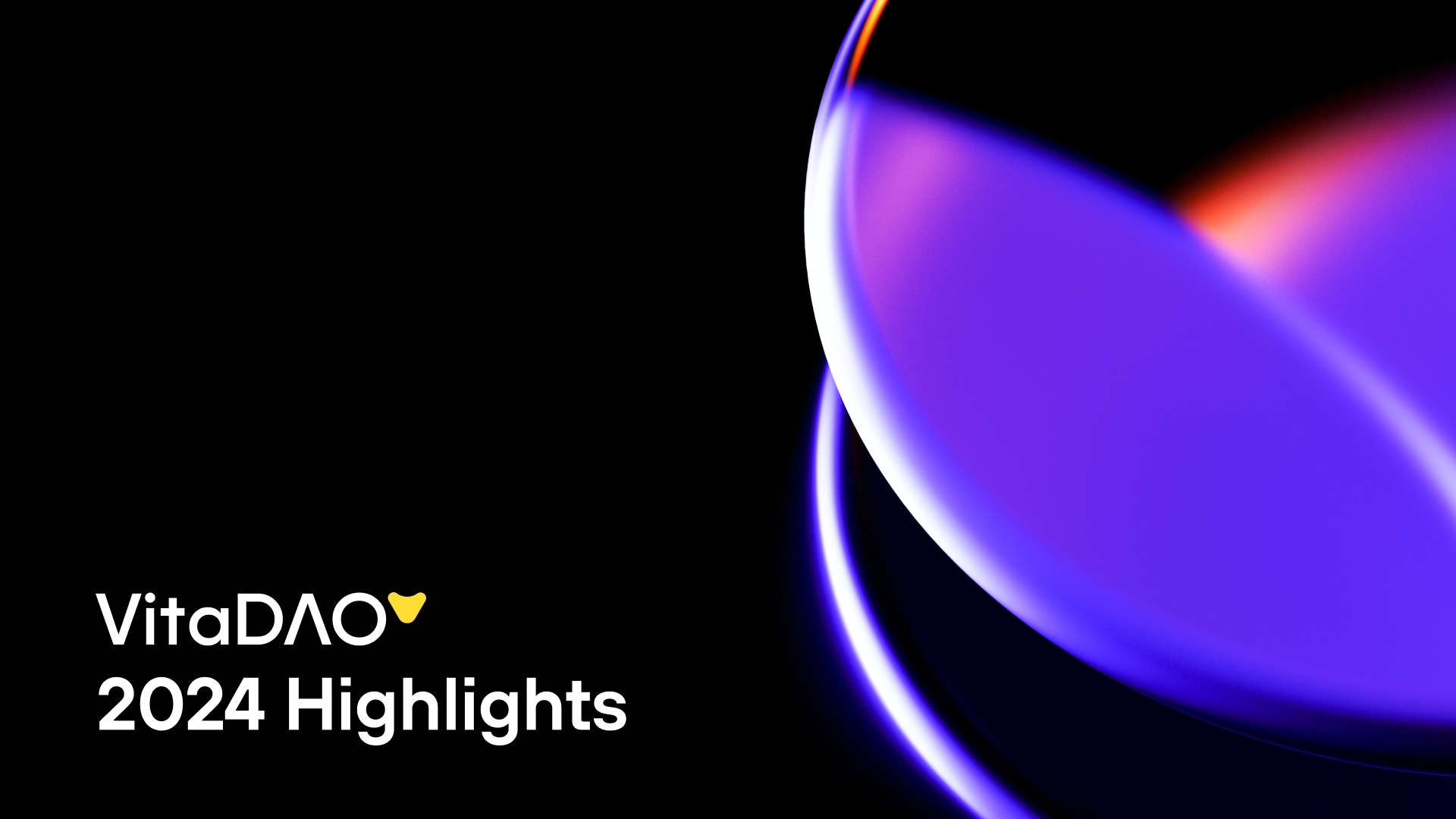