Increases in biological age correspond to the hindrances of aging, the concept symbolizing the overall health of an organism relative to its chronological age. In contrast to chronological aging, objective measurement of biological aging has been elusive: epigenetic clocks have subsequently emerged as innovative lab tests for quantifying aspects of biological aging.
What is an Epigenetic Clock?
Most commonly, the term epigenetic clock refers to a DNA methylation clock. These clocks track the presence of methyl groups, small molecules composed of carbon and hydrogen, at regions of DNA called CpG sites. Methylation patterns contribute to the regulation of gene expression and are known to shift in relation to environment, lifestyle, and aging. Researchers can use CpG methylation as a proxy to uncover patterns of gene expression in relation to aging or disease¹.
Another more complex example of epigenetic control of gene expression is histone modification. In histone modification, a few different types of chemical groups can attach to histone tails of DNA coils called nucleosomes; as such, gene expression is altered based on the type of chemical group and location of its attachment. Although histone modifications are also known to adjust with age, DNA CpG methylation is better studied in its relation to aging² ³.
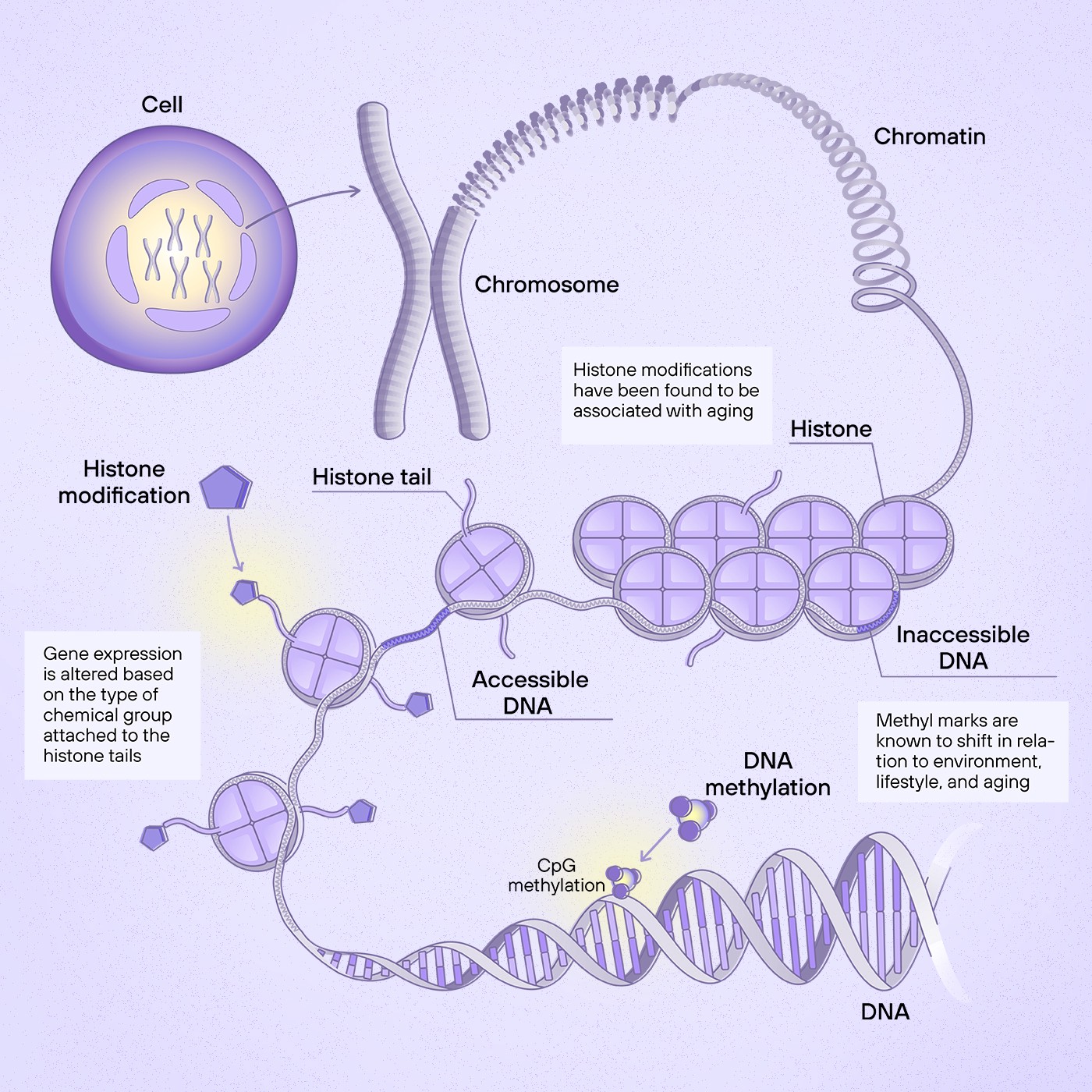
What are the Differences Between Various Clocks?
Methylation clocks have been constructed in numerous ways, and multiple versions currently exist. Clocks can differ in the number and location of sites selected, tissue types sampled, and methods used for computation⁴. As such, they can diverge in displaying different aspects of aging, enabling them to be tailored for distinct purposes. Some clocks are connected tightly to chronological age, rendering them less useful for tracking biological age but valuable for use in forensics and animal conservation⁵ ⁶. Alternatively, they can be constructed to associate with traits of aging and disease ³.
Early clocks were primarily based on correlation with chronological age. One of the first generations of clocks developed was the Hannum Clock, developed by Gregory Hannum Ph.D. et. al, which identified 71 sites predictive of age. In this clock, sex and genetic differences were determined to be primary influencers of the aging rate, with men aging 4% faster on average⁷. However, many clocks have since been developed, sampling different sites and have found various novel elements associated with aging.
“As models of human aging improve, it is conceivable that biological age, as measured from molecular profiles, might one day supersede chronological age in the clinical evaluation and treatment of patients.”
- Hannum et. al (2013)
The Horvath Clock, perhaps one of the most well-known original clocks, was developed by Steve Horvath, Ph.D., Sc.D., a prominent researcher studying human genetics and biostatistics. It utilized 353 CpG sites from multiple tissues, finding an epigenetic age close to zero for embryonic cells and age acceleration in cancer cells⁸. Horvath has since developed other clocks, including GrimAge, which is said to be accurate in predicting mortality. PhenoAge, another clock developed by Horvath along with Morgan Levine, Ph.D., was trained on established blood biomarkers and has been found to be predictive of survival, healthspan, and physical function⁹.
Both Horvath and Levine continue to research methylation clocks. Horvath recently developed a clock based on changes in multiple tissues in several mammalian species, which suggests a component of aging is evolutionarily conserved¹⁰. Meanwhile, Levine created a meta-clock by combining similarities detected in different methylation clocks. This meta-clock revealed common patterns associated with decreased metabolism, immune function, increased chromatin modifications, and autophagy dysregulation⁴.
What are the Potential Applications of Methylation Clocks?
There is still much to be explored in the research of methylation clocks, but the most obvious application is biomarkers for evaluating aging interventions. This could allow the effectiveness of a given intervention to be determined within a time frame realistic for a clinical trial. Their utility also does not need to be limited to just aging; they could similarly be tailored for quantifying the efficacy of treatments for many diseases. This could further the aim of precision medicine, allowing therapies to be tested and tailored to the treatment response of an individual.
Is Rejuvenation by Resetting the Clock Possible?
There is speculation that epigenetic markers are not only useful for measuring aging but — akin to a stopwatch — their reset might turn back the clock, reversing significant cellular aspects of aging itself¹¹ ¹². Caution is presently warranted on this topic since the research supporting it in vivo is scarce; however, there is some evidence that the alteration of epigenetic markers is associated with the restoration of youthful attributes in cells. This is supported by studies investigating epigenetic reprogramming, which can return aged cells to a stem cell state, and partial reprogramming, which according to a few reports has resulted in rejuvenated tissues¹³ ¹⁴. Additionally, a few studies, with a limited number of participants, have reported reversal of epigenetic clocks in humans undergoing different sets of pharmaceutical or lifestyle interventions¹⁵ ¹⁶.
At the same time, there is still a debate over how reliable epigenetic clocks are, and large human trials to confirm these results do not exist. There is some variance in the epigenetic age of an individual between tissue types and even between cells from the same tissue sample. Likewise, even the exact same sample can vary on repeat assessment due to lack of consistency in DNA methylation measurement; one study found a range in variance of up to 3 to 9 years in a survey of 6 different clocks¹⁷ ¹⁸. Furthermore, there are still many questions about how exactly methylation patterns result in the cell and tissue changes associated with aging¹⁹.
What are the Considerations When Purchasing Tests for Biological Age?
Healthcare, like other systems within modern societies, is trending towards decentralization and personalization, with direct-to-consumer lab tests and wearable health trackers being prime examples. Along with this trend, larger numbers of people will likely aspire to track their own health using such methods, with quite a few “biological age” tests already commercially available. Home testing of biological age may empower people to take control of their own health, but also comes with important considerations.
Although several manufacturers claim that these tests can track “age-reversal” the current evidence does not entirely support this. Still, these tests might be worthwhile, allowing an individual to incrementally trial different lifestyle interventions to determine how much impact each has on the test result, ideally translating the benefits to improved health. For example, individuals could trial different diets (adjusting macronutrients, micronutrients, caloric intake, or fasting schedules) to see what impact they might have. Other interventions within reach could focus on the amount and type of exercise, hot or cold stressors, meditation, or sleep.
Like genetic tests or other lab assays, epigenetic testing constitutes personal health information and can inform much about disease risk and personal history.
A few issues should be considered when pursuing home biological age testing. Foremost, it would be best if the variance between tests or reliability is known and reported. A high degree of variation would render the test unreliable for trialing any interventions in plausible time frames; a test that varies in reliability by a few years would probably be meaningless. An increase in testing frequency could help balance out small amounts of variability and confirm a trend. However, most of the currently available tests are priced at a few hundred dollars per test, which could make testing on a regular basis unrealistic for many people.
Additionally, having some background knowledge on the aspects of aging captured by such tests would be helpful. This type of transparency would be useful both for interpreting the results and helping to expose biases. For example, a company could sell a test along with a supplement advertised to reduce aging. The supplement might have an impact on the test, giving the appearance of reversing age, but might not have any association with aging or only correlate with a single aspect of aging like inflammation. Although inflammation plays an important role in aging, sometimes referred to as “inflammaging”, other signals could be more ominous. We cannot rule out the possibility that a test may capture a signal shared by both rejuvenation and cancer growth, like cell proliferation or loss of cell type.
Consumers should also consider privacy in addition to reliability, cost, and value. Like genetic tests or other lab assays, epigenetic testing constitutes personal health information and can inform much about disease risk and personal history. For example, both smoking history and alcohol use have been ascertained in methylation studies²⁰ ²¹. Consumers will need to determine their level of risk tolerance for privacy when submitting such data. Understanding the testing methods, reliability, and privacy terms would aid consumers in determining if such testing is appropriate and useful to them.
Final Thoughts on Biological Aging Tests
Advances in defining and measuring biological age hold promise for detecting interventions with the greatest impact on improving health. While tests are currently available to consumers, they can be pricey, and questions remain about their reliability.
Laboratory research into epigenetic clocks for measuring biological age continues to progress and appears encouraging for exploring underlying mechanisms of aging and investigating the efficacy of therapies. Someday, this type of testing might even be added to traditional clinical biomarkers of health, aiding in the realization of precision preventative medicine.
DISCLAIMER: THIS ARTICLE DOES NOT PROVIDE MEDICAL ADVICE. No material in this article is intended to be a substitute for professional medical advice, diagnosis, or treatment. The text, images, and other material contained in this article are for informational purposes only.
Lindsay Ciocco is a practicing optometrist pursuing a Master of Arts in science writing at Johns Hopkins University. She earned a Bachelor of Science in evolution and ecology, and a Doctorate of Optometry from The Ohio State University. She has an interest in writing about biotechnology, especially advances for treating ocular disease.
Victoria Padure is an illustrator and graphic designer passionate about science and longevity. With her artwork, she hopes to bring more awareness around these fields and see them thriving
Ariella Coler-Reilly is an MD Ph.D. candidate at Washington University in St. Louis studying the genetics of aging. She moonlights as a science writer and illustrator, currently working as a managing editor for the VitaDAO blog. She is passionate about public education, diversity & inclusion, and the intersection of science & web3.
References
1. Salameh Y, Bejaoui Y, el Hajj N. DNA Methylation Biomarkers in Aging and Age-Related Diseases. Frontiers in Genetics. 2020;11:171. doi:10.3389/FGENE.2020.00171/BIBTEX
2. Yi SJ, Kim K. New Insights into the Role of Histone Changes in Aging. International Journal of Molecular Sciences. 2020;21(21):1–20. doi:10.3390/IJMS21218241
3. Bergsma T, Rogaeva E. DNA Methylation Clocks and Their Predictive Capacity for Aging Phenotypes and Healthspan. Neuroscience Insights. 2020;15. doi:10.1177/2633105520942221
4. Liu Z, Leung D, Thrush K, et al. Underlying features of epigenetic aging clocks in vivo and in vitro. Aging Cell. 2020;19(10):e13229. doi:10.1111/ACEL.13229
5. Montesanto A, D’Aquila P, Lagani V, et al. A New Robust Epigenetic Model for Forensic Age Prediction. Journal of Forensic Sciences. 2020;65(5):1424–1431. doi:10.1111/1556–4029.14460
6. Barratclough A, Smith CR, Gomez FM, et al. Accurate Epigenetic Aging in Bottlenose Dolphins (Tursiops truncatus), an Essential Step in the Conservation of at-Risk Dolphins. J Zool Bot Gard. 2021;2021:416–420. doi:10.3390/jzbg2030030
7. Hannum G, Guinney J, Zhao L, et al. Genome-wide Methylation Profiles Reveal Quantitative Views of Human Aging Rates. Molecular Cell. 2013;49(2):359–367. doi:10.1016/J.MOLCEL.2012.10.016
8. Horvath S. DNA methylation age of human tissues and cell types. Genome Biology. 2013;14(10):R115. doi:10.1186/GB-2013–14–10-R115
9. Levine ME, Lu AT, Quach A, et al. An epigenetic biomarker of aging for lifespan and healthspan. AGING. 2018;10(4). Accessed March 11, 2022. www.aging-us.com
10. CONSORTIUM MM, Lu AT, Fei Z, et al. Universal DNA methylation age across mammalian tissues. bioRxiv. Published online January 19, 2021:2021.01.18.426733. doi:10.1101/2021.01.18.426733
11. Rando TA, Chang HY. Aging, rejuvenation, and epigenetic reprogramming: Resetting the aging clock. Cell. 2012;148(1–2):46–57. doi:10.1016/j.cell.2012.01.003
12. Noroozi R, Ghafouri-Fard S, Pisarek A, et al. DNA methylation-based age clocks: From age prediction to age reversion. Ageing Research Reviews. 2021;68:101314. doi:10.1016/J.ARR.2021.101314
13. Lu Y, Brommer B, Tian X, et al. Reprogramming to recover youthful epigenetic information and restore vision. Nature. 2020;588(7836):124–129. doi:10.1038/s41586–020–2975–4
14. Wang C, Rabadan Ros R, Martinez-Redondo P, et al. In vivo partial reprogramming of myofibers promotes muscle regeneration by remodeling the stem cell niche. doi:10.1038/s41467–021–23353-z
15. Fahy GM, Brooke RT, Watson JP, et al. Reversal of epigenetic aging and immunosenescent trends in humans. Aging Cell. 2019;18(6):e13028. doi:10.1111/ACEL.13028
16. Fitzgerald KN, Hodges R, Hanes D, et al. Potential reversal of epigenetic age using a diet and lifestyle intervention: a pilot randomized clinical trial. Aging. 2021;13(7):9419–9432. doi:10.18632/aging.202913
17. Sugden K, Hannon EJ, Arseneault L, et al. Patterns of Reliability: Assessing the Reproducibility and Integrity of DNA Methylation Measurement. Patterns. 2020;1(2). doi:10.1016/J.PATTER.2020.100014/ATTACHMENT/A01126CF-8D98–4563–9807–6641905A764B/MMC2.XLSX
18. Higgins-Chen AT, Thrush KL, Wang Y, et al. A computational solution for bolstering reliability of epigenetic clocks: Implications for clinical trials and longitudinal tracking. bioRxiv. Published online April 19, 2021:2021.04.16.440205. doi:10.1101/2021.04.16.440205
19. Unnikrishnan A, Freeman WM, Jackson J, Wren JD, Porter H, Richardson A. The role of DNA methylation in epigenetics of aging. Pharmacology and Therapeutics. 2019;195:172–185. doi:10.1016/J.PHARMTHERA.2018.11.001
20. Maas SCE, Vidaki A, Wilson R, et al. Validated inference of smoking habits from blood with a finite DNA methylation marker set. European Journal of Epidemiology. 2019;34(11):1055. doi:10.1007/S10654–019–00555-W
21. Liu C, Marioni RE, Hedman AK, et al. A DNA methylation biomarker of alcohol consumption. Molecular Psychiatry 2018 23:2. 2016;23(2):422–433. doi:10.1038/mp.2016.192

